The planarian is nobody's idea of a genius. A flatworm shaped like a comma, it can be found wriggling through the muck of lakes and ponds worldwide. Its pin-size head has a microscopic structure that passes for a brain. Its two eyespots are set close together in a way that makes it look cartoonishly confused. It aspires to nothing more than life as a bottom-feeder.
But the worm has mastered one task that has eluded humanity's greatest minds: perfect regeneration. Tear it in half, and its head will grow a new tail while its tail grows a new head. After a week two healthy worms swim away.
Growing a new head is a neat trick. But it's the tail end of the worm that intrigues Tufts University biologist Michael Levin. He studies the way bodies develop from single cells, among other things, and his research led him to suspect that the intelligence of living things lies outside their brains to a surprising degree. Substantial smarts may be in the cells of a worm's rear end, for instance. “All intelligence is really collective intelligence, because every cognitive system is made of some kind of parts,” Levin says. An animal that can survive the complete loss of its head was Levin's perfect test subject.
On supporting science journalism
If you're enjoying this article, consider supporting our award-winning journalism by subscribing. By purchasing a subscription you are helping to ensure the future of impactful stories about the discoveries and ideas shaping our world today.
In their natural state planaria prefer the smooth and sheltered to the rough and open. Put them in a dish with a corrugated bottom, and they will huddle against the rim. But in his laboratory, about a decade ago, Levin trained some planaria to expect yummy bits of liver puree that he dripped into the middle of a ridged dish. They soon lost all fear of the rough patch, eagerly crossing the divide to get the treats. He trained other worms in the same way but in smooth dishes. Then he decapitated them all.
Levin discarded the head ends and waited two weeks while the tail ends regrew new heads. Next he placed the regenerated worms in corrugated dishes and dripped liver into the center. Worms that had lived in a smooth dish in their previous incarnation were reluctant to move. But worms regenerated from tails that had lived in rough dishes learned to go for the food more quickly. Somehow, despite the total loss of their brains, those planaria had retained the memory of the liver reward. But how? Where?
It turns out that regular cells—not just highly specialized brain cells such as neurons—have the ability to store information and act on it. Now Levin has shown that the cells do so by using subtle changes in electric fields as a type of memory. These revelations have put the biologist at the vanguard of a new field called basal cognition. Researchers in this burgeoning area have spotted hallmarks of intelligence—learning, memory, problem-solving—outside brains as well as within them.
Until recently, most scientists held that true cognition arrived with the first brains half a billion years ago. Without intricate clusters of neurons, behavior was merely a kind of reflex. But Levin and several other researchers believe otherwise. He doesn't deny that brains are awesome, paragons of computational speed and power. But he sees the differences between cell clumps and brains as ones of degree, not kind. In fact, Levin suspects that cognition probably evolved as cells started to collaborate to carry out the incredibly difficult task of building complex organisms and then got souped-up into brains to allow animals to move and think faster.
That position is being embraced by researchers in a variety of disciplines, including roboticists such as Josh Bongard, a frequent Levin collaborator who runs the Morphology, Evolution, and Cognition Laboratory at the University of Vermont. “Brains were one of the most recent inventions of Mother Nature, the thing that came last,” says Bongard, who hopes to build deeply intelligent machines from the bottom up. “It's clear that the body matters, and then somehow you add neural cognition on top. It's the cherry on the sundae. It's not the sundae.”
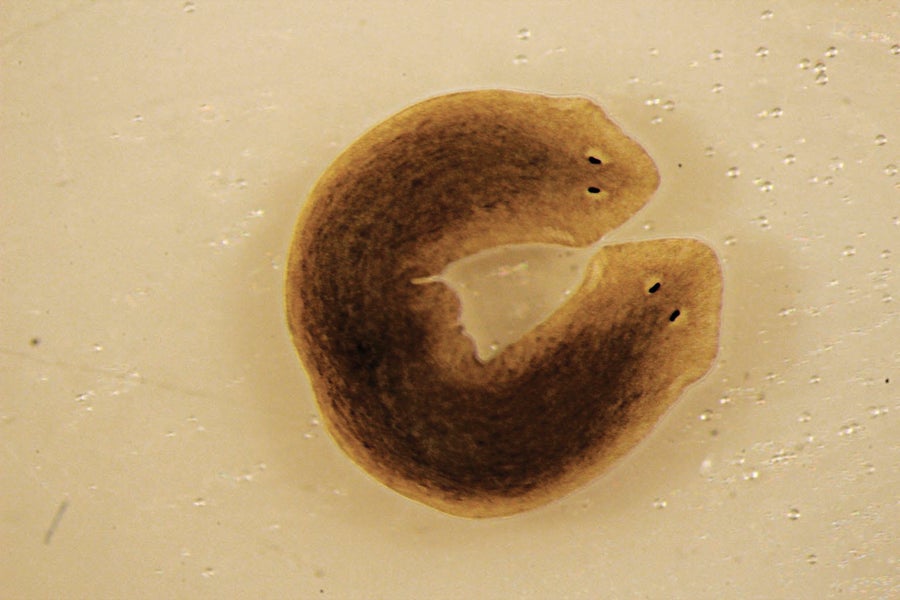
Head cells in the flatworm Dugesia japonica have different bioelectric voltages than tail cells do. Switch the voltages around and cut off the tail, and the head will regenerate a second head. Credit: Michael Levin
In recent years interest in basal cognition has exploded as researchers have recognized example after example of surprisingly sophisticated intelligence at work across life's kingdoms, no brain required. For artificial-intelligence scientists such as Bongard, basal cognition offers an escape from the trap of assuming that future intelligences must mimic the brain-centric human model. For medical specialists, there are tantalizing hints of ways to awaken cells' innate powers of healing and regeneration.
And for the philosophically minded, basal cognition casts the world in a sparkling new light. Maybe thinking builds from a simple start. Maybe it is happening all around us, every day, in forms we haven't recognized because we didn't know what to look for. Maybe minds are everywhere.
Although it now seems like a Dark Ages idea, only a few decades ago many scientists believed that nonhuman animals couldn't experience pain or other emotions. Real thought? Out of the question. The mind was the purview of humans. “It was the last beachhead,” says Pamela Lyon of the University of Adelaide, a scholar of basal cognition, who coined the term for the field in 2018. Lyon sees scientists' insistence that human intelligence is qualitatively different as just another doomed form of exceptionalism. “We've been ripped from every central position we've inhabited,” she points out. Earth is not the center of the universe. People are just another animal species. But real cognition—that was supposed to set us apart.
Now that notion, too, is in retreat as researchers document the rich inner lives of creatures increasingly distant from us. Apes, dogs, dolphins, crows and even insects are proving more savvy than suspected. In his 2022 book The Mind of a Bee, behavioral ecologist Lars Chittka chronicles his decades of work with honeybees, showing that bees can use sign language, recognize individual human faces, and remember and convey the locations of far-flung flowers. They have good moods and bad, and they can be traumatized by near-death experiences such as being grabbed by an animatronic spider hidden in a flower. (Who wouldn't be?)
But bees, of course, are animals with actual brains, so a soupçon of smarts doesn't really shake the paradigm. The bigger challenge comes from evidence of surprisingly sophisticated behavior in our brainless relatives. “The neuron is not a miracle cell,” says Stefano Mancuso, a University of Florence botanist who has written several books on plant intelligence. “It's a normal cell that is able to produce an electric signal. In plants almost every cell is able to do that.”
On one plant, the touch-me-not, feathery leaves normally fold and wilt when touched (a defense mechanism against being eaten), but when a team of scientists at the University of Western Australia and the University of Firenze in Italy conditioned the plant by jostling it throughout the day without harming it, it quickly learned to ignore the stimulus. Most remarkably, when the scientists left the plant alone for a month and then retested it, it remembered the experience. Other plants have other abilities. A Venus flytrap can count, snapping shut only if two of the sensory hairs on its trap are tripped in quick succession and pouring digestive juices into the closed trap only if its sensory hairs are tripped three more times.
These responses in plants are mediated by electric signals, just as they are in animals. Wire a flytrap to a touch-me-not, and you can make the entire touch-me-not collapse by touching a sensory hair on the flytrap. And these and other plants can be knocked out by anesthetic gas. Their electric activity flatlines, and they stop responding as if unconscious.
Plants can sense their surroundings surprisingly well. They know whether they are being shaded by part of themselves or by something else. They can detect the sound of running water (and will grow toward it) and of bees' wings (and will produce nectar in preparation). They know when they are being eaten by bugs and will produce nasty defense chemicals in response. They even know when their neighbors are under attack: when scientists played a recording of munching caterpillars to a cress plant, that was enough for the plant to send a surge of mustard oil into its leaves.
Plants' most remarkable behavior tends to get underappreciated because we see it every day: they seem to know exactly what form they have and plan their future growth based on the sights, sounds and smells around them, making complicated decisions about where future resources and dangers might be located in ways that can't be boiled down to simple formulas. As Paco Calvo, director of the Minimal Intelligence Laboratory at the University of Murcia in Spain and author of Planta Sapiens, puts it, “Plants have to plan ahead to achieve goals, and to do so, they need to integrate vast pools of data. They need to engage with their surroundings adaptively and proactively, and they need to think about the future. They just couldn't afford to do otherwise.”
None of this implies that plants are geniuses, but within their limited tool set, they show a solid ability to perceive their world and use that information to get what they need—key components of intelligence. But again, plants are a relatively easy case—no brains but lots of complexity and trillions of cells to play with. That's not the situation for single-celled organisms, which have traditionally been relegated to the “mindless” category by virtually everyone. If amoebas can think, then humans need to rethink all kinds of assumptions.
Yet the evidence for cogitating pond scum grows daily. Consider the slime mold, a cellular puddle that looks a bit like melted Velveeta and oozes through the world's forests digesting dead plant matter. Although it can be the size of a throw rug, a slime mold is one single cell with many nuclei. It has no nervous system, yet it is an excellent problem solver. When researchers from Japan and Hungary placed a slime mold at one end of a maze and a pile of oat flakes at the other, the slime mold did what slime molds do, exploring every possible option for tasty resources. But once it found the oat flakes, it retreated from all the dead ends and concentrated its body in the path that led to the oats, choosing the shortest route through the maze (of four possible solutions) every time. Inspired by that experiment, the same researchers then piled oat flakes around a slime mold in positions and quantities meant to represent the population structure of Tokyo, and the slime mold contorted itself into a very passable map of the Tokyo subway system.
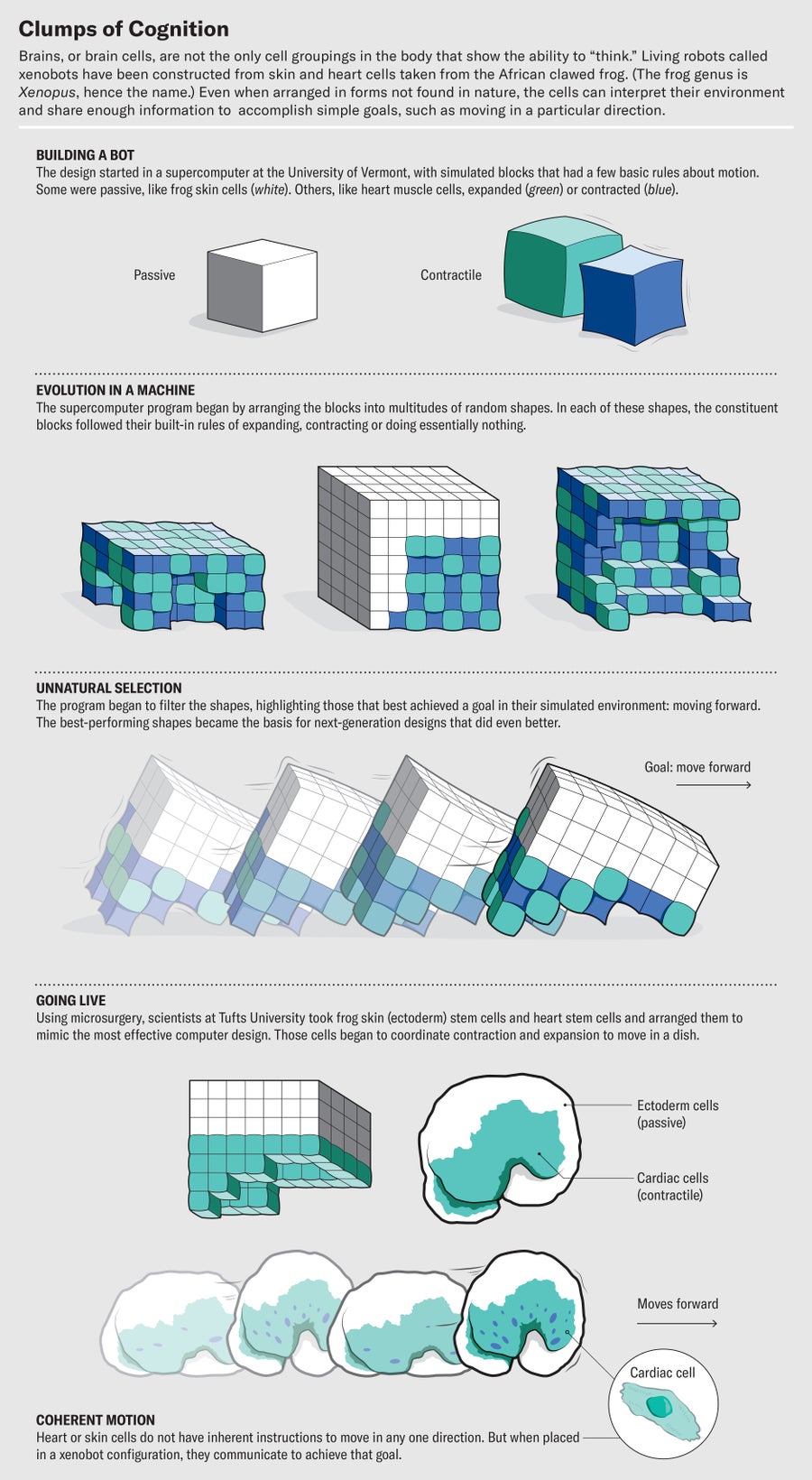
Credit: Brown Bird Design; Source: “A Scalable Pipeline for Designing Reconfigurable Organisms,” by Sam Kriegman et al., in PNAS, Vol. 117; January 2020 (reference)
Such problem-solving could be dismissed as simple algorithms, but other experiments make it clear that slime molds can learn. When Audrey Dussutour of France's National Center for Scientific Research placed dishes of oatmeal on the far end of a bridge lined with caffeine (which slime molds find disgusting), slime molds were stymied for days, searching for a way across the bridge like an arachnophobe trying to scooch past a tarantula. Eventually they got so hungry that they went for it, crossing over the caffeine and feasting on the delicious oatmeal, and soon they lost all aversion to the formerly distasteful stuff. They had overcome their inhibitions and learned from the experience, and they retained the memory even after being put into a state of suspended animation for a year.
Which brings us back to the decapitated planaria. How can something without a brain remember anything? Where is the memory stored? Where is its mind?
The orthodox view of memory is that it is stored as a stable network of synaptic connections among neurons in a brain. “That view is clearly cracking,” Levin says. Some of the demolition work has come from the lab of neuroscientist David Glanzman of the University of California, Los Angeles. Glanzman was able to transfer a memory of an electric shock from one sea slug to another by extracting RNA from the brains of shocked slugs and injecting it into the brains of new slugs. The recipients then “remembered” to recoil from the touch that preceded the shock. If RNA can be a medium of memory storage, any cell might have the ability, not just neurons.
Indeed, there's no shortage of possible mechanisms by which collections of cells might be able to incorporate experience. All cells have lots of adjustable pieces in their cytoskeletons and gene regulatory networks that can be set in different conformations and can inform behavior later on. In the case of the decapitated planaria, scientists still don't know for sure, but perhaps the remaining bodies were storing information in their cellular interiors that could be communicated to the rest of the body as it was rebuilt. Perhaps their nerves' basic response to rough floors had already been altered.
Levin, though, thinks something even more intriguing is going on: perhaps the impression was stored not just within the cells but in their states of interaction through bioelectricity, the subtle current that courses through all living things. Levin has spent much of his career studying how cell collectives communicate to solve sophisticated challenges during morphogenesis, or body building. How do they work together to make limbs and organs in exactly the right places? Part of that answer seems to lie in bioelectricity.
The fact that bodies have electricity flickering through them has been known for centuries, but until quite recently most biologists thought it was mostly used to deliver signals. Shoot some current through a frog's nervous system, and the frog's leg kicks. Neurons used bioelectricity to transmit information, but most scientists believed that was a specialty of brains, not bodies.
Since the 1930s, however, a small number of researchers have observed that other types of cells seem to be using bioelectricity to store and share information. Levin immersed himself in this unconventional body of work and made the next cognitive leap, drawing on his background in computer science. He'd supported himself during school by writing code, and he knew that computers used electricity to toggle their transistors between 0 and 1 and that all computer programs were built up from that binary foundation. So as an undergraduate, when he learned that all cells in the body have channels in their membranes that act like voltage gates, allowing different levels of current to pass through them, he immediately saw that such gates could function like transistors and that cells could use this electricity-driven information processing to coordinate their activities.
To find out whether voltage changes really altered the ways that cells passed information to one another, Levin turned to his planaria farm. In the 2000s he designed a way to measure the voltage at any point on a planarian and found different voltages on the head and tail ends. When he used drugs to change the voltage of the tail to that normally found in the head, the worm was unfazed. But then he cut the planarian in two, and the head end regrew a second head instead of a tail. Remarkably, when Levin cut the new worm in half, both heads grew new heads. Although the worms were genetically identical to normal planaria, the one-time change in voltage resulted in a permanent two-headed state.
For more confirmation that bioelectricity could control body shape and growth, Levin turned to African clawed frogs, common lab animals that quickly metamorphose from egg to tadpole to adult. He found that he could trigger the creation of a working eye anywhere on a tadpole by inducing a particular voltage in that spot. By simply applying the right bioelectric signature to a wound for 24 hours, he could induce regeneration of a functional leg. The cells took it from there.
“It's a subroutine call,” Levin says. In computer programming, a subroutine call is a piece of code—a kind of shorthand—that tells a machine to initiate a whole suite of lower-level mechanical actions. The beauty of this higher level of programming is that it allows us to control billions of circuits without having to open up the machine and mechanically alter each one by hand. And that was the case with building tadpole eyes. No one had to micromanage the construction of lenses, retinas, and all the other parts of an eye. It could all be controlled at the level of bioelectricity. “It's literally the cognitive glue,” Levin says. “It's what allows groups of cells to work together.”
Levin believes this discovery could have profound implications not only for our understanding of the evolution of cognition but also for human medicine. Learning to “speak cell”—to coordinate cells' behavior through bioelectricity—might help us treat cancer, a disease that occurs when part of the body stops cooperating with the rest of the body. Normal cells are programmed to function as part of the collective, sticking to the tasks assigned—liver cell, skin cell, and so on. But cancer cells stop doing their job and begin treating the surrounding body like an unfamiliar environment, striking out on their own to seek nourishment, replicate and defend themselves from attack. In other words, they act like independent organisms.
Why do they lose their group identity? In part, Levin says, because the mechanisms that maintain the cellular mind meld can fail. “Stress, chemicals, genetic mutations can all cause a breakdown of this communication,” he says. His team has been able to induce tumors in frogs just by forcing a “bad” bioelectric pattern onto healthy tissue. It's as if the cancer cells stop receiving their orders and go rogue.
Even more tantalizingly, Levin has dissipated tumors by reintroducing the proper bioelectric pattern—in effect reestablishing communication between the breakaway cancer and the body, as if he's bringing a sleeper cell back into the fold. At some point in the future, he speculates, bioelectric therapy might be applied to human cancers, stopping tumors from growing. It also could play a role in regenerating failing organs—kidneys, say, or hearts—if scientists can crack the bioelectric code that tells cells to start growing in the right patterns. With tadpoles, in fact, Levin showed that animals suffering from massive brain damage at birth were able to build normal brains after the right shot of bioelectricity.
Levin's research has always had tangible applications, such as cancer therapy, limb regeneration and wound healing. But over the past few years he's allowed a philosophical current to enter his papers and talks. “It's been sort of a slow rollout,” he confesses. “I've had these ideas for decades, but it wasn't the right time to talk about it.”
That began to change with a celebrated 2019 paper entitled “The Computational Boundary of a Self,” in which he harnessed the results of his experiments to argue that we are all collective intelligences built out of smaller, highly competent problem-solving agents. As Vermont's Bongard told the New York Times, “What we are is intelligent machines made of intelligent machines made of intelligent machines all the way down.”
For Levin, that realization came in part from watching the bodies of his clawed frogs as they developed. In frogs' transformation from tadpole to adult, their faces undergo massive remodeling. The head changes shape, and the eyes, mouth and nostrils all migrate to new positions. The common assumption has been that these rearrangements are hardwired and follow simple mechanical algorithms carried out by genes, but Levin suspected it wasn't so preordained. So he electrically scrambled the normal development of frog embryos to create tadpoles with eyes, nostrils and mouths in all the wrong places. Levin dubbed them “Picasso tadpoles,” and they truly looked the part.
If the remodeling were preprogrammed, the final frog face should have been as messed up as the tadpole. Nothing in the frog's evolutionary past gave it genes for dealing with such a novel situation. But Levin watched in amazement as the eyes and mouths found their way to the right arrangement while the tadpoles morphed into frogs. The cells had an abstract goal and worked together to achieve it. “This is intelligence in action,” Levin wrote, “the ability to reach a particular goal or solve a problem by undertaking new steps in the face of changing circumstances.” Fused into a hive mind through bioelectricity, the cells achieved feats of bioengineering well beyond those of our best gene jockeys.
Some of the most intense interest in Levin's work has come from the fields of artificial intelligence and robotics, which see in basal cognition a way to address some core weaknesses. For all their remarkable prowess in manipulating language or playing games with well-defined rules, AIs still struggle immensely to understand the physical world. They can churn out sonnets in the style of Shakespeare, but ask them how to walk or to predict how a ball will roll down a hill, and they are clueless.
According to Bongard, that's because these AIs are, in a sense, too heady. “If you play with these AIs, you can start to see where the cracks are. And they tend to be around things like common sense and cause and effect, which points toward why you need a body. If you have a body, you can learn about cause and effect because you can cause effects. But these AI systems can't learn about the world by poking at it.”
Bongard is at the vanguard of the “embodied cognition” movement, which seeks to design robots that learn about the world by monitoring the way their form interacts with it. For an example of embodied cognition in action, he says, look no further than his one-and-a-half-year-old child, “who is probably destroying the kitchen right now. That's what toddlers do. They poke the world, literally and metaphorically, and then watch how the world pushes back. It's relentless.”
Bongard's lab uses AI programs to design robots out of flexible, LEGO-like cubes that he calls “Minecraft for robotics.” The cubes act like blocky muscle, allowing the robots to move their bodies like caterpillars. The AI-designed robots learn by trial and error, adding and subtracting cubes and “evolving” into more mobile forms as the worst designs get eliminated.
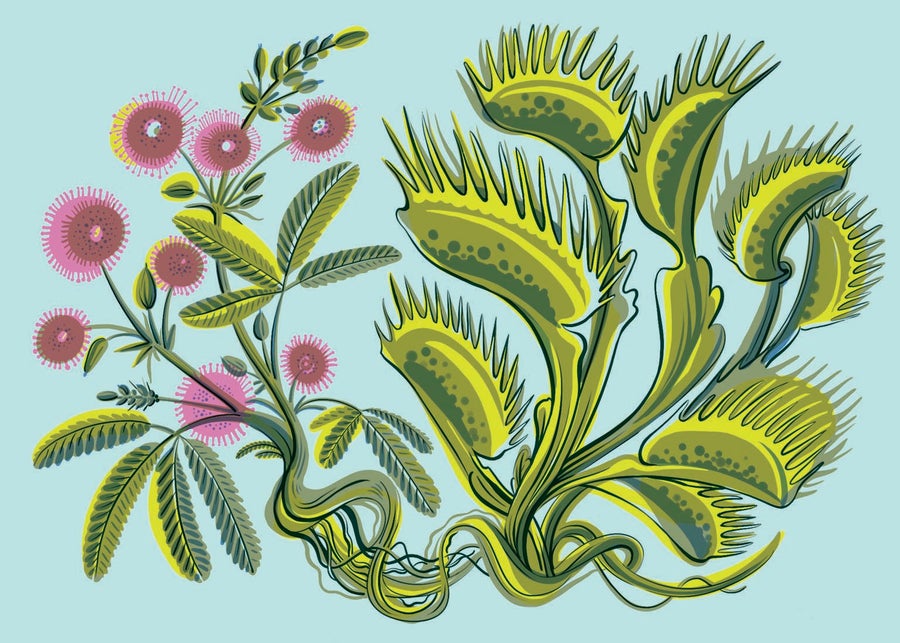
Plants use bioelectricity to communicate and take action. If you brush a sensory hair on a Venus flytrap (right), and the flytrap is wired to a touch-me-not plant (left), leaves on the touch-me-not will fold and wilt. Credit: Natalya Balnova
In 2020 Bongard's AI discovered how to make robots walk. That accomplishment inspired Levin's lab to use microsurgery to remove live skin stem cells from an African clawed frog and nudge them together in water. The cells fused into a lump the size of a sesame seed and acted as a unit. Skin cells have cilia, tiny hairs that typically hold a layer of protective mucus on the surface of an adult frog, but these creations used their cilia like oars, rowing through their new world. They navigated mazes and even closed up wounds when injured. Freed from their confined existence in a biological cubicle, they became something new and made the best of their situation. They definitely weren't frogs, despite sharing the identical genome. But because the cells originally came from frogs of the genus Xenopus, Levin and Bongard nicknamed the things “xenobots.” In 2023 they showed similar feats could be achieved by pieces of another species: human lung cells. Clumps of the human cells self-assembled and moved around in specific ways. The Tufts team named them “anthrobots.”
To Levin, the xenobots and anthrobots are another sign that we need to rethink the way cognition plays out in the actual world. “Typically when you ask about a given living thing, you ask, ‘Why does it have the shape it has? Why does it have the behaviors it has?' And the standard answer is evolution, of course. For eons it was selected for. Well, guess what? There have never been any xenobots. There's never been any pressure to be a good xenobot. So why do these things do what they do within 24 hours of finding themselves in the world? I think it's because evolution does not produce specific solutions to specific problems. It produces problem-solving machines.”
Xenobots and anthrobots are, of course, quite limited in their capabilities, but perhaps they provide a window into how intelligence might naturally scale up when individual units with certain goals and needs come together to collaborate. Levin sees this innate tendency toward innovation as one of the driving forces of evolution, pushing the world toward a state of, as Charles Darwin might have put it, endless forms most beautiful. “We don't really have a good vocabulary for it yet,” he says, “but I honestly believe that the future of all this is going to look more like psychiatry talk than chemistry talk. We're going to end up having a calculus of pressures and memories and attractions.”
Levin hopes this vision will help us overcome our struggle to acknowledge minds that come in packages bearing little resemblance to our own, whether they are made of slime or silicon. For Adelaide's Lyon, recognizing that kinship is the real promise of basal cognition. “We think we are the crown of creation,” she says. “But if we start realizing that we have a whole lot more in common with the blades of grass and the bacteria in our stomachs—that we are related at a really, really deep level—it changes the entire paradigm of what it is to be a human being on this planet.”
Indeed, the very act of living is by default a cognitive state, Lyon says. Every cell needs to be constantly evaluating its surroundings, making decisions about what to let in and what to keep out and planning its next steps. Cognition didn't arrive later in evolution. It's what made life possible.
“Everything you see that's alive is doing this amazing thing,” Lyon points out. “If an airplane could do that, it would be bringing in its fuel and raw materials from the outside world while manufacturing not just its components but also the machines it needs to make those components and doing repairs, all while it's flying! What we do is nothing short of a miracle.”